Thermochemical processing of waste to hydrogen
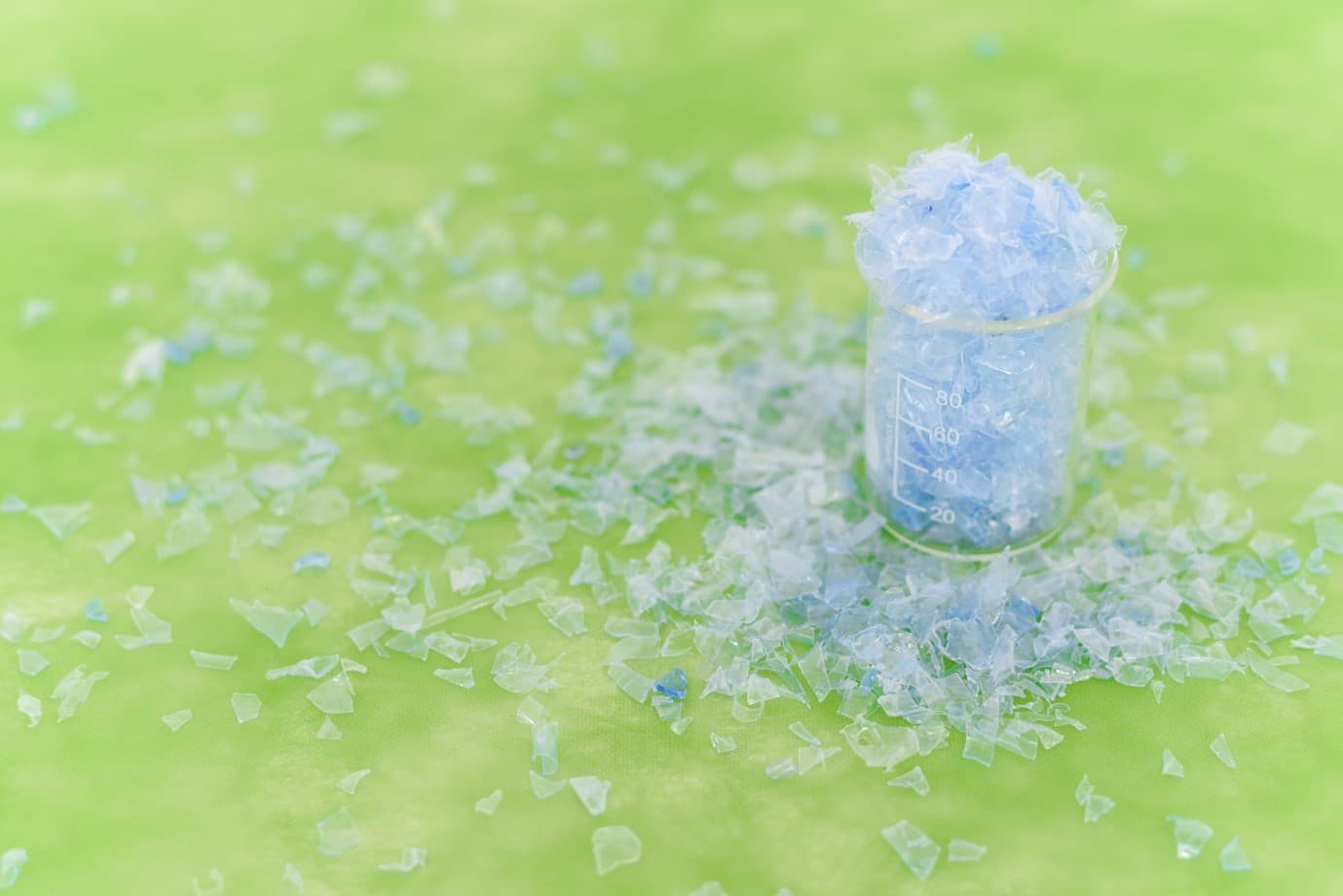
Chemcycling is gaining traction as a viable waste to hydrogen pathway. The combination of various thermolysis and purification technologies can generate high purity hydrogen from a range of wastes. This chemical recycling pathway can contribute to the drive for sustainability and decarbonisation.
Thermolysis processes such as gasification and pyrolysis convert carbon-based fuels to syngas. Syngas from waste thermolysis can be further processed via Fischer-Tropsch synthesis to methanol or liquid fuels. Alternatively, syngas can be converted to hydrogen, an emission-free energy vector.
Thermolysis can gasify virtually all organic materials ranging from municipal solid waste, biomass, sewage, manufacturing waste, plastic waste, hospital waste, agricultural and slaughter waste. Other types of mixed organic waste can also be processed if the system is appropriately engineered with respect to material selection and syngas cleaning. Wastes that contain a high proportion of PVC can be problematic because the chlorine can cause problems: poisoning catalysts and corroding steel.
In addition to flexibility with regards to feedstocks and products, pyrolysis and gasification have another advantage: the oxygen-deficient atmosphere prevents the formation of toxic oxidised pollutants, such as dioxins and furans.
The first stage is preparation of the waste. Recyclables must be removed first. The segregated waste is then fed into the first reactor stage which is generally pyrolysis. The pyrolysis reactor operates at around 750 °C in the absence of air. In pyrolysis, the feedstock is thermally decomposed into gaseous, solid, and liquid components. Pyrolysis reactor technologies can be in the form of a fluidised bed or moving bed.
Combination of pyrolysis with reforming produces very small amounts of tar. As the gases leave the pyrolysis reaction vessel, steam is added. The reforming takes place at 920 °C and tars are cracked at high temperatures and in the presence of steam to form short-chain hydrocarbon molecules.
As an additional polishing stage, a partial oxidation reactor (POX) can be used as a third thermolysis process. It is important to feed pure oxygen to the POX reactor, rather than air. This avoids NOx emissions, which cause materials selection challenges for the process equipment and contribute to air pollution.
The POX stage destroys tars and other large hydrocarbons due to the high temperature operation and the presence of a controlled amount of oxygen. In the POX reactor a controlled amount of oxygen is added – less than that would be used for complete combustion, where oxygen is added in excess. This ensures that production of carbon monoxide is favoured in preference to carbon dioxide.
Syngas leaving the exothermic POX reactor is at very high temperature. This is another benefit of using POX in the overall process. Recovery of heat from the syngas with a radiant/convective heat exchanger or direct quench improves the overall process efficiency. The heat can be used to produce steam for power generation or process heating.
Conventional syngas cleaning is performed by means of systems such as wet scrubbing which comprises a venturi scrubber followed by cooling to remove excess moisture. Typical syngas clean-up and conditioning processes include cyclone and filters for bulk particulates removal followed by wet scrubbing to remove fine particulates, ammonia, and chlorides. Solid absorbents such as activated carbon may also be required for mercury and trace heavy metal removal.
If syngas is the target, the process is now complete. If hydrogen is the target, the syngas can flow to high and low temperature water gas shift (WGS) reactors which progressively increase the ratio of hydrogen to carbon monoxide (CO) in the syngas.
To maximise the conversion of CO to hydrogen, the WGS reaction is carried out in two stages. In the first stage, a high-temperature shift (HTS) is performed at 300–450 °C, followed by a low-temperature shift (LTS) reaction operated at 180–230 °C. Steam injection can be used to optimise the reaction conditions.
The catalyst used in the HTS reactor is generally a chromium or copper promoted, iron-based catalyst. It is relatively insensitive to sulphur compounds. On the other hand, the LTS catalyst typically comprises copper and zinc and is extremely sensitive to poisoning by sulphur compounds.
If required, the syngas can be conditioned with catalytic hydrolysis to convert carbonyl sulphide to hydrogen sulphide and then remove the sulphurous gases using an acid gas removal process.
Finally, pressure swing adsorption (PSA) is used to purify the hydrogen contained in the syngas. The PSA process yields a dry, hydrogen-rich product containing 98–99.999% hydrogen with a hydrogen recovery of 70–90%.
CO and some hydrogen exit the PSA as ‘tail-gas’ which can be burned with some additional natural gas in a mixed-fuel burner system to create the energy that is required for the endothermic pyrolysis and reforming reactions.
Find out more...
Find out more about black, grey, green, blue, purple and turquoise hydrogen at the new NexantECA Training course: Hydrogen – Clean and powerful energy, helping you to understand hydrogen’s role in the energy transition. Keep an eye for our next scheuled Hydrogen training course by visiting our 2021 training calendar
The Author
Stephen B. Harrison, Principal